Principal Investigator
At a Glance
The widely discussed carbon mitigation strategy, carbon dioxide (CO2) capture and use, is often touted as a way to improve the use of fossil fuel carbon. The idea is to make vehicle fuel by chemically reducing the CO2in the exhaust stream of a fossil fuel power plant, thereby using the carbon extracted with a fossil fuel twice—once for power and once for transport. This reasoning is flawed, because the same carbon benefit can almost always be achieved more straightforwardly and at lower cost by an alternate use of the large amount of low-carbon energy required to make fuel from CO2. Only a high oil price and a high price for electric vehicles can create a domain of competitiveness for CO2 capture and use.
Research Highlight
A leading strategy for combatting climate change is CO2 capture and storage, or CCS. It is already deployed at a few coal power plants. The CO2 that results from combustion is captured with chemicals and sent into geological formations deep below ground for long-term storage. Upon hearing about CCS for the first time, laypeople and experts alike ask: If you go to the trouble of capturing CO2 at power plants, surely there is something better to do with it than to put it underground?
This is such a reasonable question! The strategy that is being sought even has a name: “Carbon Dioxide Capture and Use,” or CCU. Worldwide, chemists are seeking new ways to “activate” CO2 to make CCU more competitive. This Highlight explores the CCU economy2.
Today’s economy and the CCU economy Figure 3.3. (top panel) shows a simplified representation of today’s fossil-fuel-based energy economy, as well as the CCU economy and two alternatives. In all panels, chemically reduced carbon is removed from the subsurface as fossil fuel (red arrow), oxidized to release the energy that powers the economy (rounded rectangle), and sent to the atmosphere as CO2 for disposal (blue arrow). The sketch separates today’s economy into two sub-economies, one where energy is used centrally and the other where energy is widely distributed before use.
In the CCU economy (upper-middle panel), the blue and red arrows in the top panel are unchanged, but the passage of carbon through the economy is more complex. CO2 is captured at a centralized facility after fossil fuel is burned, then chemically reduced to a synthetic fuel in a conversion facility with the help of low-carbon energy (green dashed arrow), and the synthetic fuel is burned at a decentralized energy conversion device (e.g., in a vehicle engine). Thus, there are two power plants: one provides the CO2 (the “source plant”) and the other enables the conversion of the CO2 into synthetic fuels (the “CCU-enabling plant”). In a transaction internal to CCU, the source plant pays a “tipping fee” to the conversion facility instead of paying the government a CO2 emissions tax or paying for CO2 storage. For specificity, imagine that in the CCU system wind power transforms coal-power-plant exhaust into gasoline. The circle represents the conversion process.
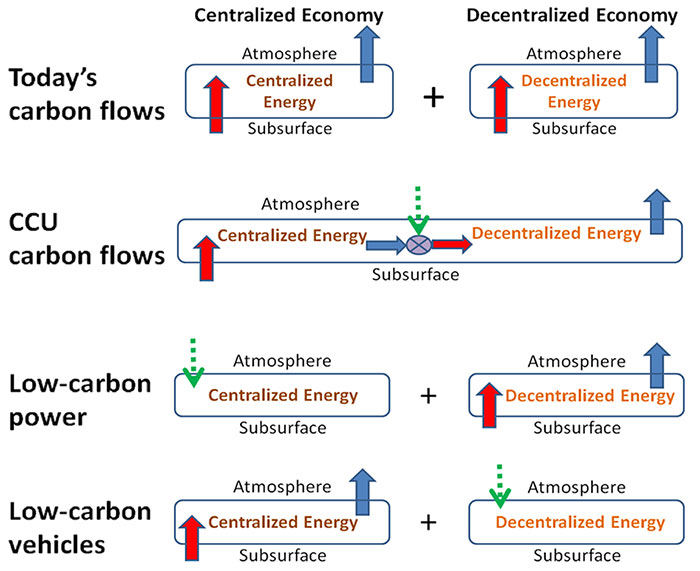
In the CCU economy (upper-middle panel), the blue and red arrows in the top panel are unchanged, but the passage of carbon through the economy is more complex. CO2 is captured at a centralized facility after fossil fuel is burned, then chemically reduced to a synthetic fuel in a conversion facility with the help of low-carbon energy (green dashed arrow), and the synthetic fuel is burned at a decentralized energy conversion device (e.g., in a vehicle engine). Thus, there are two power plants: one provides the CO2 (the “source plant”) and the other enables the conversion of the CO2 into synthetic fuels (the “CCU-enabling plant”). If the source of CO2 for CCU is a cement or steel plant, rather than a power plant, some of the arguments here are less strong, because substitution of a low-carbon alternative is less straightforward, given that carbon flows are associated not only with energy production but also with the industrial process. In a transaction internal to CCU, the source plant pays a “tipping fee” to the conversion facility instead of paying the government a CO2 emissions tax or paying for CO2 storage. For specificity, imagine that in the CCU system wind power transforms coal-power-plant exhaust into gasoline. The circle represents the conversion process.
Alas, CCU is a deeply flawed concept, primarily because there are nearly always better ways of using the low-carbon enabling energy.
Low-carbon enabling energy for CCU Somewhat more than one unit of enabling energy must be used to convert CO2 (and water) into one unit of high-value energy embedded in carbon-based liquid or gaseous fuel. How else could the enabling energy be used? Two limiting cases are presented in the lower-middle and bottom panels of Figure 3.3., respectively, where low-carbon energy substitutes exclusively for either centralized or distributed uses of fossil energy. Imagine that in the “Low- Carbon Power” system wind power enables the closing down of traditional coal plants, while the gasoline system remains unchanged. And imagine that the “Low-Carbon Vehicle” system is one where wind power enables electric vehicles, while coal power plants keep running.
All three options can become less costly than doing nothing when the CO2 price is high; the case for CCU rests on there being situations where CCU competes favorably with the other options. Comparing the CCU option and the Low-Carbon Vehicle option (bottom panel), there is a breakeven price for electric vehicles above which CCU synthetic fuels are competitive. CCU can prosper only when little progress has been made toward the electrification of vehicles and the use of biomass-derived fuel. Such a world rarely emerges in the low-carbon narratives embedded in today’s scenarios. Rather, the common view is that the electrification of decentralized energy, especially in transport, will flourish when a strong CO2 emissions constraint is imposed; sometimes, only air travel is not electrified by mid-century.
Comparing the CCU option and the Low-Carbon Power option (lower-middle panel), there is another breakeven price, the price of crude oil (and therefore crude-oil-derived vehicle fuels), above which CCU synthetic fuels are competitive. Thus, CCU requires both a high oil price and a high price for electric vehicles. Either constraint can be the one that limits the competitiveness of CCU. In a highly idealized schematic model, CCU must be less expensive than both (crude-oil fuel minus coal power) and (electric vehicles minus wind power).
CO2 capture cost We cannot neglect the substantial investment required to capture the CO2 at the source plant. In a circumstance particularly favorable to CCU, but surely a niche market, the source power plant has been a CCS plant, and thus has already paid the capture costs, but for some reason its access to storage has ended.
Delay time CCU is sometimes presented as a CO2 storage strategy. It is not. An important variable is the delay time: the length of time between the capture event at the source plant and the moment of CO2 emission when the CCU fuel is used. A delay time of several decades or longer can occur in principle, but only if the captured carbon becomes embedded in a long-lived product like a plastic bench or a steel pipe.
Enhanced oil recovery The CCU panel of Figure 3.3. represents only uses of CO2 where it is transformed chemically. CO2 chemically unchanged is used in the food system and for cleaning, but by far its largest use is in the oil industry for “enhanced oil recovery (EOR),” where CO2 is injected into old oil fields to promote the extraction of additional oil. Nearly all of the CO2 brought to the oil field for EOR remains there when oil production ceases, trapped in geological formations. EOR today leaves about one carbon atom behind in the oil field for each carbon atom in the produced oil.
EOR could be modified so that much more carbon is left behind. To the disappointment of the many champions of CO2 reuse as a pathway to hydrocarbons, EOR may be the only climate-significant version of CCU.
2 In the second half of 2016, I served on a task force that wrote a report for the US Department of Energy. Entitled “Task Force Report on CO2 Utilization and Negative Emissions Technologies,” it was submitted to Secretary of Energy, Ernest J. Moniz, on December 13, 2016. It is online at https://energy.gov/seab/downloads/final-reporttask-force-co2-utilization , where the task force participants and a DOE “Assessment” of the report are also found. This Highlight explores an issue that was left unresolved in our report. It is a work in progress.
3 If the source of CO2 for CCU is a cement or steel plant, rather than a power plant, some of the arguments here are less strong, because substitution of a low-carbon alternative is less straightforward, given that carbon flows are associated not only with energy production but also with the industrial process.
4 In a highly idealized schematic model, CCU must be less expensive than both (crude-oil fuel minus coal power) and (electric vehicles minus wind power).
Acknowledgments
I particularly wish to thank Nate Lewis for his insistence that CCU was being overvalued and his patience while I struggled to make the argument my own. Along the way, exchanges especially with Arun Majumdar, but also with Sally Benson, Emily Carter, Mike Ramage, and Eric Toone, were essential.